The "fingerprints" of the Havre eruption
As final step to solve the Havre puzzle, we studied the experimental particles and ash grains of the "4 phi" sieve fraction (i.e. with a grain size between 63 µm and 125 µm) under the scanning electron microscope (SEM). We used the shape analysis software PARTISAN to quantify the particle shapes in 2D. Each sample set contained 50 or more particles, and each particle is characterized by 18 different shape parameters so it makes sense to apply statistical methods for comparisons. More concrete, we used a sequence of F- tests, t-tests and equivalence tests:
- F-tests for testing the homogeneity of variances between two data sets
- t-tests for verifying significant differences between two data sets
- equivalence tests (e-tests) for testing two data sets for statistical equivalence
We applied the above mentioned tests by following the subsequent schematic protocol:
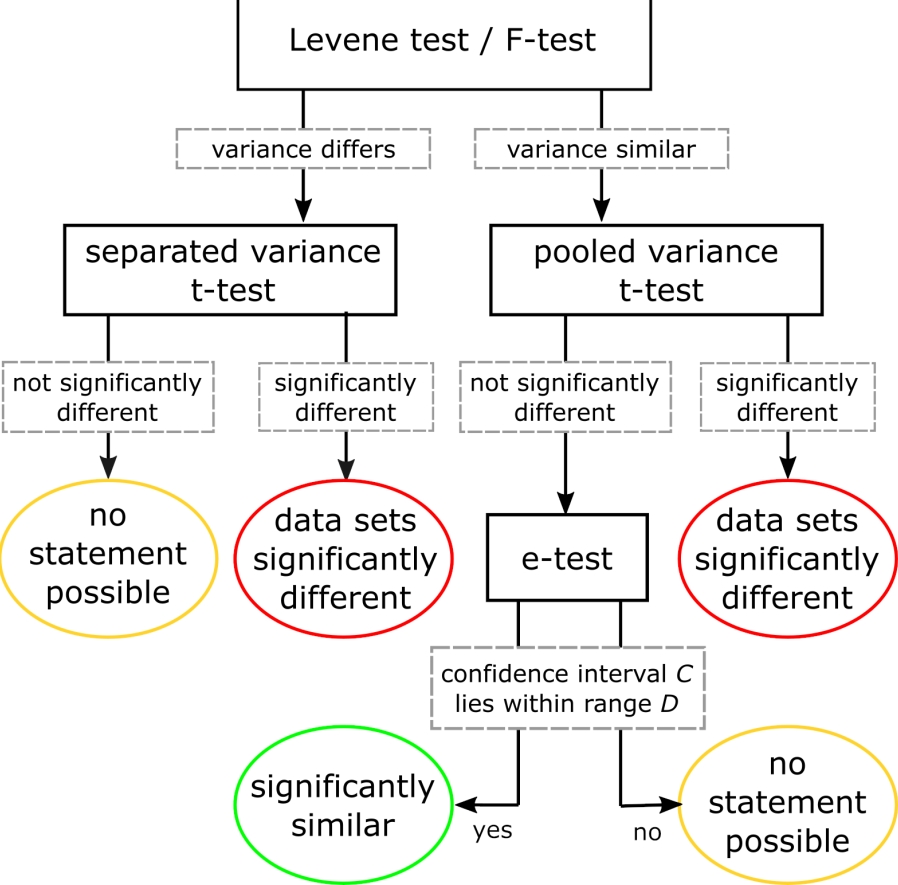
Schematic protocol to test two data sets for significant differences and similarities. (Figure adapted from Dürig et al. (2020) [1].)
We morphometrically analyzed curvi-planar (blocky) Havre ash grains (labelled "Nat") and compared them with the following experimental samples:
- DG: particles produced in dry stress-induced runs and collected on the experimental area ("G" stands for "ground")
- IU: IFCI particles produced and collected via IFCI "U-tube" runs
- IG: IFCI particles produced in IFCI runs with open configuration and collected on the experimental area ("G" stands for "ground")
- IW: IFCI particles produced in IFCI runs with open configuration and collected within water droplets collected on the ceilings and walls ("W" stands for "water")
The following table summarizes the results based on these multiple statistical comparisons:
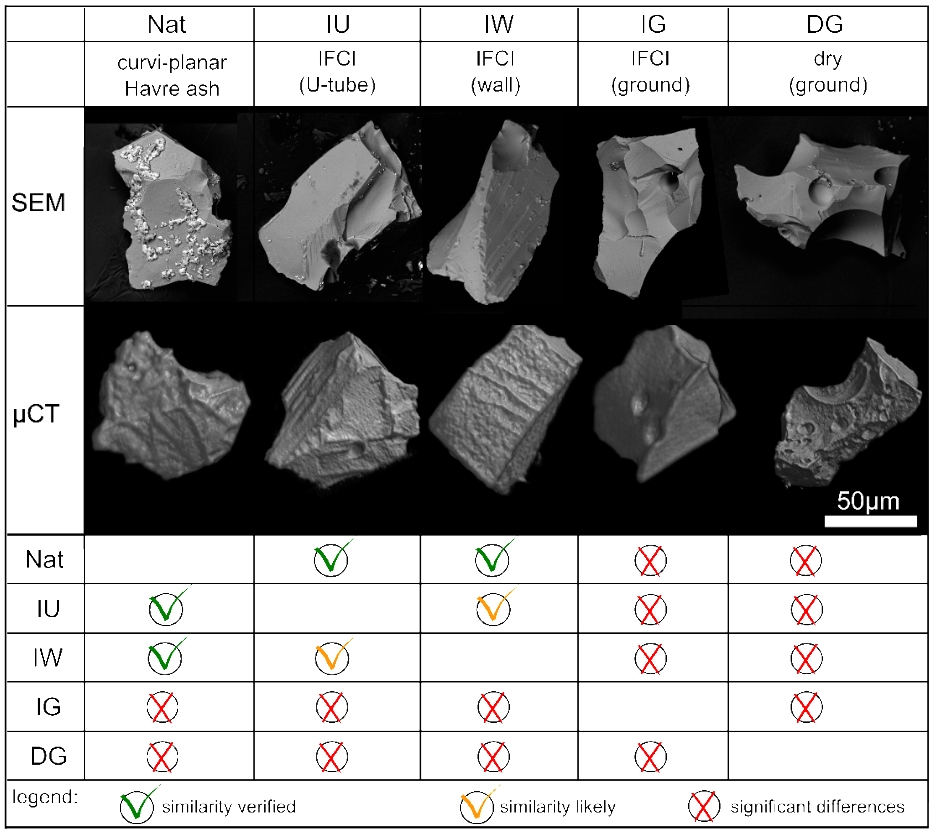
Results of morphological analyses. (Figure is adapted from Dürig et al. (2020) [1], please check there for picture with higher resolution and for more detailed discussion.)
According to our previous considerations, these were exactly the samples for which we would expect that they contain the highest percentage of IFCI particles.
We thus have identified IFCI as the fragmentation mechanism responsible for the generation of curvi-planar Havre ash. Since this ash type represents the bulk of Havre ash retrieved on the sea floor, we can conclude that IFCI was the dominant mechanism during the ash generating phase of the 2012 Havre eruption.
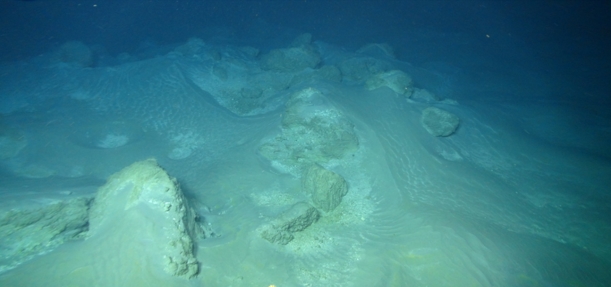
Scattered pieces of evidence on the sea floor: Havre ash, pictured during the 2015 deep-sea diving mission. (Picture by courtesy of James D.L. White).
Notes:
The Havre ash project was funded by a Marsden grant, New Zealand.
The lines of thought above are based on considerations detailed in Dürig et al. (2020) [1] if not cited otherwise. Please let me know if you have any comment or suggestions for changes. My email address is: tobi[_at]hi.is
References and Annotations
[2]: T. Dürig, D. Mele, P. Dellino, B. Zimanowski, Comparative analyses of glass fragments from brittle fracture experiments and volcanic ash particles. Bulletin of Volcanology 74 (3), 691-704 (2012)